|
 | 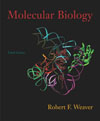 Molecular Biology, 3/e Robert F. Weaver
Professional ResourcesProfessional ResourcesChapter 1
Wakayama,T, A.C.F. Perry, M. Zuccotti, D.R. Johnson, and R. Yanagimachi (1998). Full-term development of mice from enucleated oocytes injected with cumulus cell nuclei. Nature 394:369-74. This landmark paper describes the cloning of mice from somatic cells. Chapter 5
Femino, A.M., F.S. Fay, D. Fogarty, and R.H. Singer (1998). Visualization of single RNA transcripts in situ. Science 280:585-90. The authors refined the FISH method by making oligodeoxynucleotide probes with five fluorochromes per molecule and hybridizing these to actin mRNAs within cells. The method was so sensitive that it could detect single molecules of RNA. Chapter 6
Zhang, G., and S.A. Darst (1998). Structure of the Escherichia coli RNA polymerase subunit amino terminal domain. Science 281:262-6. This paper shows that the dimerization domain of the polymerase subunit contains two ?-helices that interact with the corresponding helices in the other monomer. This places the sites that interact with other polymerase subunits on one face of the ?2 dimer. Chapter 9
Kielkopf, C.L., S. White, J.W. Szewczyk, J.M. Turner, E.E. Baird, P.B. Dervan, and D.C. Rees (1998). A structural basis for recognition of A?T and T?A base pairs in the minor groove of B-DNA. Science 282:111-114. An A-T base pair differs only subtly from a T-A base pair in the minor groove, yet the two can be distinguished by a synthetic polyamide. This paper presents x-ray crystallographic results that explain this phenomenon. Chapter 10
Thomas, M.J., A.A. Platas, and D.K. Hawley (1998). Transcriptional fidelity and proofreading by RNA polymerase II. Cell 93:627-37. This paper shows that TFIIS, which stimulates the 3'÷5' nuclease activity of RNA polymerase II, also stimulates replacement of misincorporated nucleotides during rapid RNA synthesis. Chapter 11
Wieczorek, E., M. Brand, X. Jacq, and l. Tora (1998). Function of TAFII-containing complex without TBP in transcription by RNA polymerase II. Nature 393:187-91. This paper describes transcription of class II genes by complexes lacking TBP. Chapter 13
LeRoy, G., G. Orphanides, W.S. Lane, and D. Reinberg (1998). Requirement of RSF and FACT for transcription of chromatin templates in vitro. Science 282:1900-04. This paper identifies the minimal factor requirements@ for activator-dependent transcription on chromatin templates in vitro. Chapter 14
Tacke, R., M. Tohyama, S. Ogawa, and J.L. Manley (1998). Human Tra2 proteins are sequence specific activators of pre-mRNA splicing. Cell 93:139-148. This paper identifies the human counterpart of Tra2, the sex-determining splicing factor in Drosophila. The human protein does not determine sex, but it is a sequence-specific splicing activator that probably helps govern cell-specific splicing.
Li, H., C.R. Trotta and J. Abelson (1998). Crystal structure and evolution of a transfer RNA splicing enzyme. Science 280:279-84. The authors compare the structure of an archaeal enzyme to that of a eukaryal enzyme. Chapter 15
Håkansson, K., A.J. Doherty, S. Shuman, and D.B. Wigley (1997). X-ray crystallography reveals a large conformational change during guanyl transfer by mRNA capping enzymes. Cell 89:545-53. This paper compares two crystal structures of the GTP-bound form, and a GMP-bound form, of a viral capping enzyme. These structures suggest a large conformational change in the enzyme during the capping reaction.
Birse, C.E., L. Minvielle-Sebastia, B.A. Lee, W. Keller, and N.J. Proudfoot (1998). Coupling termination of transcription to messenger RNA maturation in yeast. Science 280:298-300. This paper shows that mutations in factors involved in clipping at the polyadenylation site in yeast CYC1 pre-mRNA prevented proper transcription termination.
Hirose, Y. and J.L. Manley (1998). RNA polymerase II is an essential mRNA polyadenylation factor. Nature 395:93-6. This paper demonstrates that RNA polymerase II is required, even in the absence of transcription, for polyadenylation in vitro. This supports the hypothesis that polymerase II helps recruit the factors needed for cleavage and polyadenylation.
Dantonel, J.-C., K.G.K Murthy, J.L. Manley, and L. Tora (1997). Transcription factor TFIID recruits factor CPSF for formation of 3' end of mRNA. Nature 389:399-. This paper presents data that support the hypothesis that TFIID recruits CPSF, which then associates with RNA polymerase II until it reaches the cleavage/polyadenylation signal. Chapter 16
Mackle, G.A. (1998). Ribonuclease E is a 5'-end-dependent endonuclease. Nature 395:720-3. Degradation of RNA by the E. coli ribonuclease E is inhibited by cyclization of the substrate, by hybridization of an antisense oligonucleotide to the 5'-end of the substrate, or just by addition of a triphosphate to the 5'-end of the substrate. These findings suggest that ribonuclease E requires a substrate RNA with a free 5'-end. Chapter 17
Pestova, T.V., S.I. Borukhov and C.U.T. Hellen (1998). Eukaryotic ribosomes require initiation factors 1 and 1A to locate initiation codons. Nature 394:854- . This paper presents evidence that ribosomal preinitiation complexes lacking eIF-1 and -1A can recognize and bind to the cap-proximal region of an mRNA, but cannot reach the initiation codon. Chapter 18
Lin, L., G.N. DeMartino and W.C. Greene (1998). Cotranslational biogenesis of NF-kB p50 by the 26S proteasome. Cell 92:819-28. This paper shows that two proteins, p50, and p105 can be made from the same mRNA. p50 is made when the growing polypeptide is clipped by the 26S proteasome. On the other hand, p105 is the result of failure of this cotranslational processing event.
Green, R., C. Switzer, and H.F. Noller (1998). Ribosome-catalyzed peptide-bond formation with an A-site substrate covalently linked to 23S ribosomal RNA. Science 280:286-9. This paper shows that a 23S rRNA with an aminoacyl-tRNA analog covalently attached to G2553 can undergo the peptidyl transferase reaction with a peptidyl-tRNA analog in the ribosomal P site. This places G2553 close to the A site.
Nitta, I., Y. Kamada, H. Noda, T. Ueda, and K. Watanabe (1998). Reconstitution of peptide bond formation with Escherichia. coli 23S ribosomal RNA domains. Science 281:666-9. This paper shows that a fragment (region V) of 23S rRNA has peptidyl transferase activity. The activity is lower than that of intact 23S rRNA, but is stimulated by a separate RNA molecule containing 23S rRNA region VI. Chapter 19
Lodmell, J.S. and A.E. Dahlberg (1997). A conformational switch in Escherichia. coli 16S ribosomal RNA during decoding of messenger RNA. Science 277:1262-9. This paper presents evidence for a conformational switch in 16S rRNA, involving a change in base-pairing. The switch affects tRNA binding and mRNA decoding.
Joseph, S., B. Weiser, and H.F. Noller (1997). Mapping the inside of the ribosome with an RNA helical ruler. Science 278:1093-8. Noller and colleagues have used anticodon stem-loop analogs with iron(II) attached to their 5'-ends to generate hydroxyl radicals that cleave whatever rRNA is nearby. By varying the lengths of the probes, and placing them in either the P or A site, these workers have been able to map the locations of sites in all three rRNAs relative to the P and A sites.
Wilson, K.S. and H.F. Noller (1998). Mapping the position of translational elongation factor EF-G in the ribosome by directed hydroxyl radical probing. Cell 92:131-9. Wilson and Noller attached Fe(II) to 18 different sites in EF-G, then detected rRNA clipping by the hydroxyl radicals generated by the iron ion. This procedure located the rRNA regions near each of the 18 sites in EF-G.
Saks, M.E., Sampson, J.R. and J. Abelson (1998). Evolution of a transfer RNA gene through a point mutation in the anticodon. Science 279:1665-70. This paper shows that a point mutation in the anticodon of a tRNA can change not only its codon recognition, but its charging specificity. This could help to explain the origin of isoaccepting species of tRNA.
Ban, N., B. Freeborn, P. Nissen, P. Penczek, A. Grassucci, R. Sweet, Frank, J., P.B. Moore, and T.A. Steitz (1998). Cell 93:1105-15. A 9 Å resolution x-ray crystallographic map of the large ribosomal subunit. This analysis of the ribosome confirms the map based on electron microscopy, but also reveals structures that are likely correspond to double-helical RNAs. Chapter 20
Korolev, S., J. Hsieh, G.H. Gauss, T.M Lohman, and G. Waksman (1997). Major domain swiveling revealed by the crystal structures of complexes of E. coli Rep helicase bound to single-stranded DNA and ADP. Cell 90:635-47. The crystal structures of Rep helicase bound to DNA, or to DNA and ADP show Rep in two forms which differ from each other in a large change in comformation of one of the Rep domains. Such conformation shifts may be important in Rep movement along the DNA.
Doublié, S., S.Tabor, A.M. Long, C.C. Richardson, and T. Ellenberger (1998). Crystal structure of a bacteriophage T7 DNA replication complex at 2.2 Å resolution. Nature 391:251-8. The authors crystallized the T7 DNA polymerase complexed with DNA and a nucleoside triposphate. The crystal structure suggests how the correct nucleotides are selected for incorporation into DNA.
Redinbo, M.R., L. Stewart, P. Kuhn, J.J. Champoux, and W.G.J. Hol (1998). Crystal structures of human topoisomerase I in covalent and noncovalent complexes with DNA. Science 279:1504-13. The crystal structure of this human topoisomerase complexed with DNA shows how the enzyme clamps onto DNA. Chapter 21
Blasco, M.A., H.-W. Lee, M.P. Hande, E. Samper, P.M. Lansdorp, R.A. Depinho, and C.W. Greider (1997). Telomere shortening and tumor formation by mouse cells lacking telomerase RNA. Cell 91:25-34. The authors generated knockout mice lacking the gene encoding the RNA component of telomerase. The telomeres of these mice shortened by almost 5 kb per generation and were lost by the fourth generation. At this point, chromosomal aberrations also appeared.
Bodnar, A.G., M. Ouellette, M. Frolkis, S.E. Holt, C.-P. Chiu, G.B. Morin, C.B. Harley, J.W. Shay, S. Lichtsteiner, and W.E. Wright (1998). Extension of life-span by introduction of telomerase into normal human cells. Science 279:349-52. Normal human cells lack telomerase activity and have a finite lifespan before they senesce and die. This lifespan can be extended by adding telomerase to the cells.
Guenther, B., R. Onrust, A. Sali, M. O=Donnell, and J. Kuriyan (1997). Crystal structure of the d´ subunit of the clamp-loader complex of E. coli DNA polymerase III. Cell 91:335-45. The d´ subunit of the clamp loader is homologous to the catalytic subunit, g. From the crystal structure of the d´ subunit, we infer that the g subunit is C-shaped and that nucleotides bind to the inner surface of the C. Chapter 22
Hiom, K and Gellert, M. (1997). A stable RAG1-RAG2-DNA complex that is active in V(D)J cleavage. Cell 88:65-72. This paper shows that RAG1 and RAG2 bind specifically to a DNA containing recombination signal sequences. Depending on the divalent metal provided, this complex will either nick the DNA or form hairpins at the signal sequence.
Eggleston, A.K., A.H. Mitchell, and S.C. West (1997). In vitro reconstitution of the late steps of genetic recombination in E. coli. Cell 89:607-17. The authors have used purified RuvA, RuvB, and RuvC proteins to reconstitute an in vitro system that can process a recombination intermediate formed from DNA and RecA protein.
Melek, M., Gellert, M., and D. C. van Gent (1998). Rejoining of DNA by the RAG1 and RAG2 proteins. Science 280:301-3. This paper shows that the RAG proteins can reverse the cleavage at recombination signal sequences (RSSs) by joining an RSS DNA end to a non-RSS DNA end, forming a hybrid joint.
Agrawal, A., Q.M. Eastman, and D.G. Schatz (1998). Transposition mediated by RAG1 and RAG2 and its implications for the evolution of the immune system. Nature 394:744-51. This paper shows that the RAG proteins can act as a transposase, transposing a piece of DNA flanked by RSSs to another, target DNA.
Cousineau, B., D. Smith, S. Lawrence-Cavanagh, J.E. Mueller, J. Yang, D. Mills, D. Manias, G. Dunny, A.M. Lambowitz, and M. Belfort. (1998). Retrohoming of a bacterial group II intron: Mobility via complete reverse splicing, independent of homologous DNA recombination. Cell 94:451-62. This paper shows that a bacterial group II intron can transpose to a DNA target site by reverse splicing, in the absence of functional RecA. |
|
|